My Work With Vacuum Tubes At Bell
Laboratories By John R. Pierce (c) (Reprinted from
SMEC (now SMECC) "Vintage
Electrics" Volume #3 issue #1 - 1991) |
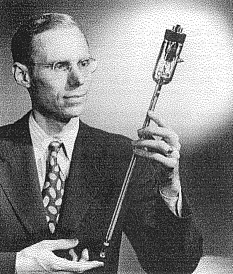 |
John Pierce Holding An Early Traveling
Wave Tube.
|
The Early Days
The date of my starting work at Bell Laboratories was to be September
1936. My parents, through a sort of general good will, and perhaps in
celebration of my receiving my doctorate and getting a job, sent me on a
trip through Europe during the summer. This was a slow journey by today's
standards, by train and small steamer, bicycling in England and traveling
by rail in France, Italy and Germany. On my return, I went back to
California to see my mother and father and to collect my few belongings,
and then on to New York, where I was to work at 463 West Street, in
Manhattan.
For two years, until I was married in 1938, I shared apartments within
walking distance of work with Chuck Elmendorf (Charles Halsey Elmendorf
III). Chuck had received an M.S. degree from Caltech; like me he had come
to work at Bell Labs.
Working at Bell Laboratories was a novel and bewildering experience. My
first boss, Dick Mendenhall, had been a favorite student of Millikan's at
Caltech. He was at that time a very handsome man, though his feet had been
severely damaged in a high-voltage accident. I saw something angelic, and
perhaps a little aloof in his features, but he treated me warmly and
invited me to his home in Summit, New Jersey.
I had a high regard for Mendenhall, but he gave me little guidance.
Neither did Mervin Kelly, not yet president of -Bell Laboratories but
somewhere way up the line. I met him at the Laboratories shortly after I
got there. Someone I didn't remember having seen before stopped me in the
hall and
asked what I was doing. I was suspicious and asked who he was. "Mervin
Kelly," he replied.
Mendenhall's direct boss was Ray Wilson, a jolly and agreeable man who,
like Kelly and all above Mendenhall, had an office high in the main Bell
Laboratories building at 463 West Street. All who worked on vacuum tubes
of Mendenhall's rank and below were in the "Biscuit Building"(a
structure built for and once used by the National Biscuit Company), just
north of the main building.
The Biscuit Building had two floors and a basement. On the upper floor,
experimental vacuum tubes were built by an organization directed by Vick
Ronci. He had a few engineers and innumerable craftsmen. There were
offices and office laboratories, separated by glass partitions, on the
south and west sides of the ground floor. In the center was a large space
for laboratory work.
I did my first work in this central space. What did I do? My account must
be based partly on my memory of remote days, and partly on the titles of
internal technical memoranda, called Memoranda for File, that I wrote
frequently during most of my years at Bell Labs.
I received little guidance except that I was to do research on vacuum
tubes. I was told of some work by Philo Farnsworth, and of a
high-frequency oscillator with no hot cathode that he had made.
Farnsworth was an ingenious inventor who never prospered largely. His
image dissector was an ingenious, almost distortionless television pickup
tube that was used for some years for scanning movie film for television
broadcast. Unlike Zworykin's Iconoscope, the image dissector didn't
involve storage, and it was not sensitive enough to pick up live scenes
with reasonable lighting.
I finally visited Farnsworth's laboratory, no doubt with others, and I
took back with me one of his oscillator tubes, which I tested and came to
understand. It wasn't of any practical value. More important, I saw the
electron multipliers that he incorporated in his image dissector and in
other devices. These seemed to me to be crude and unduly complex in
construction.
So did the magnetically focused electron multipliers the Myron Glass, a
colleague at Bell Laboratories, had built. It was Myron Glass who said,
truly, "Nature abhors a vacuum tube," a remark later attributed
to me.
Wasn't there a way to design a simple, effective electrostatically focused
multiplier? To do this, one needed a way to find where electrons would go
in a system of odd-shaped electrodes. The conventional approach would have
been to find the electric field, analytically (impractical) or
experimentally in an electrolytic tank, and then to compute the electron
trajectories (impractical in those pre-computer days).
At this time I came to know Bill Shockley, a new employee who was making
the rounds of various parts of the research department. His effect on
vacuum-tube research was electrifying, in his work with others as well as
with me. It was because of him that a practical means for designing
electron multipliers was worked out. At this point I really don't remember
whose idea it was; it may well have been Shockley's. Certainly, he had a
lot to do with the construction.
Electric potential in a two-dimensional system of electrodes is analogous
to physical height. An electode at a given potential is analogous to a
level surface. Moreover, the height of a stretched sheet of thin rubber
supported by "electrodes" of various heights is closely but not
exactly analogous to the electric potential between actual electrodes of
the same shapes.
In this rubber sheet analogy, the electric potential (height) and the
electric field (slope) appear before ones very eyes. And, even better, by
rolling ball bearings down the stretched rubber one can find very nearly,
and certainly nearly enough, where electrons will go in the analogous
electric fields. With this tool, it was easy to find a common shape for
succeeding opposed and staggered electrodes at progressively higher
potentials that would focus the secondary electrons from each electrode
effectively on that of the next higher potential.
The electrode shapes that I worked out proved very effective in the
photomultipliers that the tube shop on the second floor constructed for
me. In 1941 I finally published a paper on this electrostatically focused
photomultiplier with a colleague, R. C. Winans, who had made an improved
structure. Electron multipliers of similar design are used today. A patent
was applied for. Similar work had been done about the same time at RCA. I
don't know who got the patent.
In the course of my work on electron multipliers, I became concerned with
the noise in the output of these devices. Shockley worked out a theory. I
simplified it. We published a paper on this in the March, 1938 issue of
the Proceedings of the IRE.
As a part of an internal review of this work on noise, Thornton Fry, head
of the mathematics department at Bell Labs, and a very eminent and useful
industrial mathematician, objected to the description of the noise as a
signal with an infinitely broad, flat power spectrum. He felt that this
was mathematically unsound. He had published work on shot noise in which
the properties of the output circuit, with limited bandwidth, were an
integral part of the expression for noise. This avoided the to him
mathematically unsound idea of a fluctuation of infinite bandwidth. But,
Shockley's approach, really not new, gave the same answer as Fry's and was
less cumbersome and specialized. Fry appeared not to understand this. This
reinforced in me a distrust of authority, even sound authority.
I soon found that there wasn't any use in the Bell System for a better
photomultiplier. Broad band coaxial cable systems capable of transmitting
hundreds of telephone conversations or a television signal were just
coming into being. There was a great need for amplifier tubes with high
transconductance (the ratio of the current change at the plate to the
voltage change at the grid) and low input and output capacitance. I
thought that electron multipliers might be useful in producing such tubes.
Others had the same idea, and a few experimental tubes were produced and
marketed, but were not really successful.
The electron multiplier could be used to increase the transconductance by
producing several electrons for one, but this also increased the output
current. Ahead of the electron multiplier one needed a means for
controlling electron flow that would give a large transconductance per
unit current. In the conventional cathode-grid arrangement invented by Lee
De Forest, there
was an inescapable limit to this ratio, a limit due to the thermal
velocities of electrons leaving the cathode.
What about deflecting a beam of electrons past a sharp edge? Could the
limitation be overcome in this way? The answer proved to be yes, but I
didn't know enough physics to give a sound general treatment of the
problem. Shockley came to the rescue, and introduced me to Liouville's
theorem, which says that the density of particles in phase space (the
coordinates of phase space are position and momentum) is constant. If we
regard the motion of electrons as governed by the electric (and magnetic)
fields in which they move, and disregard interactions of one electron on
others, this applies to electrons in vacuum tubes, and the coordinates are
the three position coordinates and the three velocity coordinates.
The derivation of Liouville's theorem that Shockley gave me used
Hamilton's equations, about which I knew nothing, but I found that the
theorem could be derived easily from Newton's laws of motion, and I
suppose that's how Liouville derived it.
On October 13, 1937 Shockley and I issued a joint memorandum, Maximum
Attainable Current Densities and Deflection Type Tubes, in which we
described this work, which led to the same results that David Langmuir had
derived by somewhat different means and published about that time. I
published a paper, Limiting Current Densities in Electron Beams in the
Journal of Applied Physics in October, 1939.
Once I understood that it should be possible to attain a higher ratio of
transconductance to current in a deflection tube than in a conventional
tube using a cathode and grid, I set about to make a good deflection tube.
The idea was new to me, but I found later that it was an old idea.
The tubes I made turned out to have a substantial transconductance without
any electron multiplication, and low input and output capacitance as well.
Maybe a deflection tube without any following electron multiplier would be
useful.
In order to make a better tube, I needed a well-focused electron beam of
several milliamperes at a voltage of a couple of hundred volts.
Conventional electron gun designs were unsuitable.
The usual approach was to settle on the shapes of an electron-emitting
cathode and plausible accelerating electrodes, and to try to calculate
where the electrons would go-or, simply to build the structure and see
what happened. But, how was one to find a good cathode and electrode
structure? And, how was one to calculate the electron paths near the
cathode, where the fields were in part determined by the space charge of
the electrons themselves?
Several sorts of electron flow were known; the appropriate calculations
had been made. These were electron flow between an infinite parallel plane
cathode and an infinite parallel plane anode, flow between a cylindrical
cathode and a central cylindrical anode, and flow between a spherical
cathode and a central spherical anode. None of these was physically
feasible.
It occurred to me that one might realize a part of such flow, a
cylindrical beam between parallel anode and cathode, a wedge-shaped flow
between a segment of a cylinder and another segment of a cylinder, or
conical flow between two spherical caps. I found out that this could be
done by using electrodes outside of the beam shaped so that they would
fool the electrons in the beam into thinking that they were part of a
larger planar, or cylindrical, or spherical flow. At the anode one could
simply cut a hole to let the electrons pass. This would act as an electron
lens of calculable properties.
I published this work in a paper, Rectilinear Flow in Electron Beams in
the Journal of Applied Physics in August of 1940. Before that, I had given
a paper at either an IRE or an American Physical Society meeting. The
structure (or structures) I had devised became known as the Pierce gun,
widely used in all sorts of electron devices.
One other problem remained in deflection tubes-the limitation in current
density through beam spreading due to the charge in the electron beam. On
April 22, 1938 Ray W. Sears and I issued a Memorandum on this subject. An
elaboration of this work went into my book Theory and Design of Electron
Beams, published by Van Nostrand in 1949. I don't know whether or not I
published a paper on the subject.
What about deflection tubes? After a year or so of work, the ratio of
transconductance to capacitance was almost as good as that of the pentodes
that were made when I had started, but pentodes continued to improve.
Further, it became apparent that the transit time of electrons in a vacuum
tube had an important bad effect when such tubes were used in negative
feedback amplifiers, which were essential in coaxial cable systems.
Deflection tubes, with their long transit time, and electron multipliers,
which also had a long transit time, would be useless in the amplifiers
needed. That was the end of my work on multipliers and the deflection
tubes to which that work led. What was left of all this work?
I had learned to understand noise, in photomultipliers and other devices.
I had become acquainted with Liouville's theorem and its implications for
electron devices. I had invented an electron gun that was to have great
importance in many devices, including the traveling-wave tubes used in
communication satellites. I had made subsequently useful calculations on
the effect of-space charge in electron beams. And, I had come to have a
high regard for Bill Shockley, who was good and helpful to me, and was
often good fun as well.
Memories Of Microwave Tubes
Sometime after I came to Bell Labs J. 0. McNally became my direct boss.
Canadian by birth, a large man, good humored, he took a more direct
technical interest in what I did than Mendenhall had. Still, what I
actually did seemed entirely up to me.
My work on electron multipliers resulted in an excellent device for which
there was no use in the Bell System. My efforts to make high
transconductance tubes through electron multiplication and deflection
tubes came to nothing but a liberal education and knowledge that would be
useful later. I had also tried some other unsuccessful approaches.
Somehow, I became involved with microwaves, which seemed to be the coming
thing for broad-band long-distance communication. Later this work was to
be turned to wartime radar, but when I first visited Harald Friis's
laboratory at Holmdel, New Jersey, the emphasis was entirely on
communication. What was lacking was satisfactory microwave tubes.
When I began my work, the only microwave source I had was a triode that
would barely oscillate at a wavelength of 20 cm. This tube was mounted in
a waveguide (a cylindrical metal tube) about four inches in diameter. This
waveguide would not transmit 20 cm waves, but it would transmit 10 cm
waves, the second harmonic of the 20 cm oscillator. A little second
harmonic leaked out, and this I used as a source of microwave power for my
experiments.
The titles of my Memoranda for File indicate to me that I started to think
about microwave devices in the latter part of 1938. A memorandum titled A
Magnetron Amplifier puzzles me; I don't have the slightest idea what it
may have been.
By then, Varian had invented the klystron, and Hansen of Stanford had made
an analysis of its operation. Si (Simon) Ramo, who had received his Ph. D.
from Caltech at the same time that I did, had gone to General Electric.
There he and Hahn had studied the operation of klystrons, and had worked
out a useful theory of waves on electron streams to explain their
operation.
Might not the klystron be a useful amplifier? Arthur Samuel, who had been
at Bell Laboratories for some years before I arrived, thought so. He built
some ingenious klystron amplifier tubes that were ultimately used in the
first experimental inter-city microwave system, that linking Boston and
New York. Like other klystrons, these used a long, narrow electron beam,
and operated at a voltage of over a thousand volts.
I regarded 300 volts as more reasonable, and set out to build an amplifier
which would work at that voltage. Such a klystron amplifier would have to
incorporate closely-spaced pairs of grids in its input and output
resonators.
Designing such a tube called for an analysis different from that used by
Hansen and by Ramo and Hahn. Happily, Llewellyn and Peterson of Bell Labs
(I came to know both well) had published equations for ac fluctuations on
an electron stream flowing between parallel planes. These equations
related the velocity and current fluctuations at the second plane to the
velocity and current fluctuations at the first plane. They included the
effect of a voltage fluctuation between the two planes; this I did not
need.
Benham, a British worker, had published similar equations, but I found
that he had somehow omitted a term that gave the effect of bunching due to
velocity modulation-the very heart of klystron operation.
Armed with these equations, I set out to design a low voltage klystron
amplifier. I realized that the bunching and hence the gain would be most
effective if the beam was slowed down in the drift space between the input
and output cavities, so I put a low-voltage grid between these.
The result was an amplifier with a gain of around 10 db. My boss, J. 0.
McNally, was impressed. By that time Bell Laboratories had become involved
in wartime, or pre-wartime, radar work, and he wanted to try the tube as
an amplifier at the front end of a radar receive. I calculated how noisy
it should be, and felt sure that it would be much too noisy. Nonetheless,
I made a portable power supply and packaged the amplifier so that it could
be
carried easily to a Bell Labs radar operating somewhere out in the
country- not at Holmdel. The amplifier improved the performance of the
radar, which was much noisier than it should have been. Nonetheless, I
knew that the amplifier wouldn't help a good radar, and I managed somehow
to discourage further attempts at its exploitation.
This was easier because of a mistake I made in using the amplifier.
Accidentally, I made the grid between the input and output cavities
negative rather than positive, so that it reflected electrons back through
the input cavity. The tube oscillated like mad, and one could get on
unprecedented 100 milliwatts from the input cavity.
Further, the frequency could be changed by tuning one cavity, and the
frequency could be changed by around 20 megahertz simply by changing the
negative voltage of the reflecting electrode. This proved useful in
automatic tuning, to make the receiver track fluctuations in transmitter
frequency.
I immediately made a tube with one resonator with one pair of grids and a
negative reflecting electrode or reflector. This became the 707A, which
was used in all 10 cm or S Band American radars. It came to be called the
McNally tube.
I don't claim to have invented the reflex klystron; I stumbled on it. A
tube described earlier by Ramo was pretty close. Perhaps Varian or Hansen
had patented the device. The virtue of the reflex klystrons we produced at
Bell Laboratories for radar use was that they were simple, low voltage
devices that worked well.
After the 707A, we set out to make an X Band klystron, one that would work
at a wavelength of 3 cm. McNally felt that a tube with a glass envelope
and an external resonator, a scaled-down version of the 10 cm tube, would
be practical. We tried to make such a tube, but never produced one that
was any good. I thought we would have to make a tube with an internal
resonator. So did Gerry Shepherd (W. G. Shepherd), who had somehow been
assigned to work with me.
Tubes with internal resonators had been made at Stanford, but they were
high-voltage devices of complex construction that looked like the work of
a German machinist. Indeed, I found out that they were. What we needed was
a low voltage tube that was simple and cheap to produce.
Some years earlier there had been a passing fad for vacuum tubes with
metal envelopes. The Bell Labs tube department had acquired machinery for
fabricating such tubes. Metal tubes proved inferior to tubes with glass
envelopes, and our machinery for making them was stored away, gathering
dust.
At my urging, Gerry Shepherd set out to design a really satisfactory metal
klystron with an internal resonator. I felt that this was a job beyond the
imaginative skills of the engineers on the second floor who fabricated
tubes. Gerry learned about the capabilities of the machines for
fabricating
metal tubes, and he sat down at the drafting board and designed the X Band
oscillator himself. Years later he told me that he had felt this work a
little beneath his dignity, but he didn't object at the time.
The tube, the 723A, was a tremendous success, and inexpensive to
fabricate. It was used in all American X Band radars. It came to be known
as the Pierce-Shepherd tube.
The success of this X Band oscillator led us to build a similar metal
oscillator for use at a wavelength of 7.5 cm. Here we ran into trouble.
The electronic tuning didn't work right. Eventually we found that the
trouble was caused by reflected electrons getting clear back to the
cathode region, being reflected by the cathode, and going through the
resonator a second time. We remedied this by a change in the reflector
electrode that cause the
returned beam to spread out. By the end of 1943 we had a satisfactory
tube.
I then set out to make a K Band reflex klystron, that would oscillate at a
frequency of 1.25 cm. In this tube the cavity was so small that it was
tuned by the expansion of a metal rod heated through bombardment by
electrons. The tube worked, but was never used. I had jumped to an
erroneous conclusion.
I had assumed that the high current density necessary in a K Band tube
would preclude the use of grids, and I designed a tube without grids that
operated at 750 volts. Victor Neher, a cosmic-ray physicist then working
at the MIT Radiation Laboratory, was smart enough to calculate the grid
temperature. He found that in a 300-volt tube of plausible design the
grids would get red hot, but radiation of heat would keep them from
melting. A tube following his design, but built at Bell Laboratories,
became the standard K Band tube of the War years, and the years
thereafter.
By altering the resonator and adding grids, I turned my 1.25 centimeter
tube into a .625 centimeter oscillator which George Mueller used at
Holmdel in some measurements of rain attenuation. By this time I was
concerned mainly with traveling-wave tubes, and I made no more of these
oscillators. When they wore out, the tube development department tried,
unsuccessfully at first, to duplicate them. Eventually an excellent
engineer produced an excellent tube. But that was all after the war, when
tube work had moved to the new Murray Hill Laboratories.
After the war, Gerry Shepherd and I published a long paper in the Bell
System Technical journal, telling all we knew and all we had done
concerning reflex klystrons. Gerry went away to the University of
Minnesota, where, after a distinguished career in electronics, he became a
vice president. I turned to other matters.
It seems appropriate to mention that Vic Neher, whose K Band klystron had
beat mine out, did another important piece of work that affected work at
Bell Laboratories. Around July, 1943 Jack Morton, who then worked for me,
and I went the MIT Radiation Laboratory to see Neher's 10 centimeter
triode amplifier. The secret of success was the very fine grid and the
very small
cathode-to-grid spacing, about a thousandth of an inch. In Neher's tube
this spacing was changed a little in tuning the internal input resonator.
I was much impressed by this result, but I felt that a practical tube
would have to have a fixed cathode-to-grid spacing. I felt that this could
be attained by attaching the cathode to a ceramic disc with a hole in it
and grinding the cathode surface flush with the ceramic surface. Then a
taut tungsten grid wound on a flat tungsten washer could be spaced from
the cathode by a thin metal shim.
I suggested to Jack Morton, who then worked with me, that he try to do
this. He succeeded magnificently, on his own, when I was no longer his
boss. He set up a spotlessly clean shop for producing this tube, which
became known as the Morton triode. Morton triodes were used in preference
to traveling-wave tubes in the first Bell System transcontinental
microwave
repeater system.
During the war I had profited greatly from the work of others. I was in
touch with work in England as well as in America. From Britain I received
regularly the classified reports of the CVD (Committee for Valve
Development). The minutes of this committee described in some detail work
in various laboratories, industrial as well as government. At no other
time have I felt so close to the unfolding of important work.
From October 27, 1944 through December 23 of that year, a colleague, Homer
Hagstrum, and I visited British tube laboratories for the U. S. Navy, and
for our benefit as well. Hagstrum was engaged in work on cavity
magnetrons, whose large pulsed power had made microwave radar feasible. I
was known chiefly for my work on reflex klystrons, but I had other
interests on this trip. I had read about the traveling-wave tube, which
went beyond ideas that I had been exploring.
I note among the Memoranda that I wrote one dated August 7, 1941, Negative
Attenuation of Space Charge Waves. Sergei Schelkunoff had calculated the
attenuation of the space charge waves described by Hahn and Ramo in case
the
material outside of the electron beam was lossy or dissipative. He found a
wave with a negative attenuation, that is, a growing wave, which he
thought nonsensical. I must have summarized his work without understanding
its implications. Years later, the resistive-wall amplifier was invented
independently, a device that made use of this forgotten phenomenon. I
missed an opportunity.
I worked out the action of a circuit with a slow electromagnetic wave on
electrons with the velocity of the wave, but I didn't think of any
circuits that could be fabricated easily. I didn't build anything. And, in
my analyses, I did not go on to investigate the effect back on the circuit
of
an electron beam which had been bunched by the action of a slow wave
having a velocity near to that of the electrons. I was looking for some
novel type of microwave vacuum tube, but I didn't find it.
What I didn't find for myself, I found in a CVD report. This was the
traveling-wave tube, invented by Rudolf Kompfner. When Hagstrum and I were
in England in 1944, I met Kompfner himself. The traveling-wave tube was to
be the focus of my activities, and of those of many others, until I
abandoned work on vacuum tubes.
Traveling-Wave Tubes
Work on traveling-wave tubes occupied most of my time from 1945 until
around 1955- a period of 13 years. Today, when vacuum tubes survive almost
entirely as extremely high-power devices, and solid-state devices serve
almost all low-power and moderate-power functions, the traveling-wave tube
is still used in some communication satellites as a transmitter producing
around 10 watts.
The inventor of the traveling-wave tube was Rudi (Rudolf) Kompfner. He was
born in Austria, he emigrated to England, and on December 27, 1951 he came
to Bell Laboratories. He was my very dear friend. You can read his account
of the invention in his book, The Invention of the Traveling-Wave
Tube-that is, if you can find a copy. A continuing theme of the book is
that Rudi not only speculated; he built things, and he made measurements,
looking for the expected and sometimes finding the unexpected. The very
high gain of the traveling-wave tube was unexpected.
Rudi was taken out of detention as an enemy alien in 1941, and he was, as
he said, more or less drafted into the physics department of the
University of Birmingham, where Boot and Randall had invented the
multicavity magnetron, the centerpiece of microwave radar. He was,
however, not assigned to work on magnetrons; rather, he was told to
develop a klystron amplifier with a noise figure that would be better than
that of existing radar receivers.
In early 1944 Kompfner was transferred to a microwave tube research group
at Oxford, and that is where I met him on either November 15 or 16, 1944.
1 had already heard of his work through CVD reports.
Kompfner had created a device in which a slow electromagnetic wave acted
on a stream of electrons, bunching them, and the bunched electron stream
in turn increased the amplitude of the electromagnetic wave as it traveled
along. Kompfner analyzed the process as a series of steps the effect of
the wave on the electrons, the effect of the electrons on the wave, and so
on,
back and forth. His young colleague, Joseph Hatton, summed the resulting
series and showed that there would be an exponential growth of power along
the helix.
The various analyses that I had done of electron flow made it easy for me
to formulate the behavior of the traveling-wave tube in terms of waves.
Part of the initial excitation of the signal went into one growing wave,
which increased exponentially and provided the output power.
The first reference to such an analysis I can find is a Memorandum titled
Electronic Analysis of Traveling Wave Amplifier-II, dated November 30,
1945. I wonder, what happened to part 1? Other analyses followed in 1946
and 1947. In 1946 a popular piece about traveling-wave tubes appeared in
the Bell Laboratories Record, and in February 1947 I published a
theoretical paper in
the Proceedings of the IRE.
During this period I had made traveling-wave tubes, and they worked,
giving gains of tens of db. This was at a time when Kompfner had become
somewhat disillusioned with his invention, and worked for a while along
other lines. He used to say that he had invented the traveling-wave tube,
but that I had discovered it.
Certainly, I saw the value of the traveling-wave tube the very moment I
learned of it. Kompfner had seen the tube chiefly as a low-noise
amplifier. I saw it chiefly as a broadband amplifier. At that time I had
visions of extremely broad-band digital microwave signals, and the tube
seemed the
answer to my dreams. I was only partly right.
Although traveling-wave tubes have been used to amplify very broad-band
signals, they are chiefly used for signals with a few tens of megahertz
bandwidth. Aside from the high gain and good efficiency of traveling-wave
tubes, a chief advantage is that the circuits that shape and limit the
bandwidth are outside of the tube itself, and can thus have an
electrically and mechanically good design, and can be adjusted easily.
I understood very well that a helix had negligible loss in the backward
direction, and that if the tube had gain in the forward direction it was likely
to oscillate. I calculated the effect of loss in the helix on the gain of
the tube and found that a lot of loss would reduce the gain only a little.
So, I made my tubes lossy. Further calculations showed that it would be
best to put loss near the middle of the helix only, thus, in effect,
dividing the tube into input and output regions, and this I did.
In my early work I was much helped by Les (Lester M.) Field, who found
traveling-wave tubes more interesting than the magnetrons he had been
working on, and joined me in my work. After a few years he went to
Stanford and established traveling-wave tube work there; he later went to
Caltech, and thence to Hughes.
Others joined with me in my work; Nelson Wax, Bill Hebenstreit, Art
Hollenberg. By the time we moved the work from West Street to the Murray
Hill Laboratories, around 1949, Sid (Sidney) Millman and I, as joint
"subdepartment heads" had a considerable group working on tubes,
and mostly on traveling-wave tubes of one sort or another. The group
included Al Clogston, an able physicist who later, with Millman, turned to
work more directly in his field, and Cal Quate and Ping King Tien, two of
Les Field's students from Stanford.
Rather than trying to give a completely coherent account of what I and
those associated with me did in our work on microwave tubes, I shall
select a few topics only.
In my early work on traveling-wave tubes, I learned a lot of things not to
do. Here was a wonderful device that should be cherished and improved. I
wanted to excel or supplant it. I thought at first that I would combine
the
efficiency of the magnetron with the amplifying properties of the
traveling-wave tube. Nothing came of this except wasted time, on the part
of myself and others. Traveling-wave magnetron amplifiers were pursued
elsewhere for many years, with indifferent success. One of the most
pronounced features of electron flow in crossed electric and magnetic
fields is instability, fine in oscillators but deadly in amplifiers.
In late 1947 Bill Hebenstreit and I made an astonishing invention. This
was the double-stream amplifier, a device in which two electron beams
interact with one another to provide a wave of growing amplitude without
any circuit. How wonderful to get away from the circuit! We thought that
our idea would revolutionize microwave amplification.
It didn't. No circuit was needed for amplification, but one had to put a
signal on the electron streams at the input end and take an amplified
signal off the electron streams at the output. This necessitated coupling
circuits to two electron streams rather than one, a difficult feat. The
double-stream amplifier is a member of a large class of devices and
inventions-wonderfully ingenious, and good for nothing.
I should note that Andrew Haeff invented the double-stream amplifier at
RCA at about the time we invented it at Bell Laboratories. I don't know
who got the patent.
How did Bill Hebenstreit and I invent the double-stream amplifier? We
stumbled on it in trying to understand the effect of the Maxwellian
distribution of velocities in an electron stream. We started with a
simplified case we could analyze-an electron stream consisting of
electrons with two distinct velocities. We found waves that increased with
distance. Happily, we didn't try to treat the velocity distribution by in
infinite series and take the first terms, as Bohm and Gross did. Had we
done so, we would have found their dispersion relation, an erroneous
expression now long forgotten.
During the course of my work on microwave amplifiers, I strayed into other
useless sidetracks. A late one was the easitron, the product of Larry (L.
R.) Walker and me. In the easitron, the electron beam swept past an array
of equally spaced parallel wires stretched across a metal tube. A wave
grew like mad at a frequency near that at which the wires were a
half-wavelength long.
Later, I tried to incorporate quantum effects into expressions for the
operation of traveling-wave tubes and related devices. The results may
have been correct, but they were irrelevant.
The relevant and important problems were improvements of the
traveling-wave tube itself. One vital matter was to provide a useful
theory of the operation of the tube. This I accomplished step by step.
In my first analysis I assumed that the only field acting on the electrons
was that of the electromagnetic wave that would travel on the helix in the
absence of the electron stream. But, a bunched electron stream would
produce some electric field even inside a solid metal tube. So, I included
the effect of space charge, or passive modes. Later, I included the effect
of transverse field components.
What about the helix that carried the electromagnetic wave? Here, the
chief parameters were the wave velocity, the square of the field strength
per unit power, and the passive modes. I approximated the helix by a
cylinder which conducted in a spiral direction only; this worked out
pretty well. I later found that Franz Ollendorf, a German physicist who
emigrated to Israel, had published this analysis, and I was careful to
give him credit. We exchanged a few letters.
Some later theories of traveling-wave tubes set out to solve the partial
differential equations of an electron beam surrounded by an
electromagnetic
circuit of slightly greater diameter. This is a complex approach. While it
can provide numerically correct results, it gives little insight. Various
rather simple phenomena are inextricably mixed up in the overall numerical
answer.
One important problem in traveling-wave tubes was noise. My early
theoretical work made possible an approximate calculation of the noise for
a given structure. In the early fifties, Dean Watkins, a student of Les
Field's at Stanford, made an amazing invention, the velocity jump
amplifier. By accelerating the electron beam in a sequence of steps over
the proper distances before it entered the helix, he produced a
traveling-wave tube with an unprecedentedly low noise figure.
This intrigued me. I jumped at the correct conclusion that beyond the
electron gun, the noise in the electron steam could be represented as a
pair of space-charge waves. The noise would have a component that was a
fluctuation of velocity, and another component that was a fluctuation of
density or current. In a beam at constant potential, each of these noise
components varies periodically along the beam, the velocity noise being
highest where the current noise is lowest, and the current noise being
highest where the velocity noise is lowest. To get the best noise figure,
there must be an optimum ratio of velocity noise to current noise at the
beginning of the helix.
It occurred to me that in some sense the total noisiness of both
components together might be constant along the electron beam, however the
beam might be accelerated or decelerated. Showing that this was so was
beyond my mathematical capability, but not that of Neville Robinson, a
physicist at the Clarendon Laboratory at Oxford who spent several summers
at Bell Labs.
Neville understood matrices, which had baffled me, and proved the
necessary theorem. I published a paper on the result in the Journal of
Applied Physics of August, 1954.
A quite different sort of noise plagued early traveling wave tubes. They
exhibited noisy oscillations at frequencies of a few megahertz. I showed
theoretically that such oscillations could be caused by positive ions.
Chap (C. Chapin) Cutler had found the oscillations, and had attributed
them to the correct cause. He eliminated the oscillations by getting a
higher vacuum, and by pulling the remaining ions out of the helix by
lowering the potential of the collector electrode. But, this caused
trouble because secondary electrons from the collector went back through
the helix.
Tinkering with the magnetic focusing field near the collector cured this.
The variation of gain with signal level was important. I made a sensible
guess at the maximum output by asking what the calculated output would be
were the electron beam completely bunched. Non-linear calculations seemed
too much for me. Ping King Tien eventually made computer calculations of
high-level operation. Chap Cutler built a huge, demountable, low-frequency
(around 100 megahertz) traveling-wave tube in which he could observe and
measure the behavior of the electrons at high levels.
There were other problems associated with traveling-wave tubes. One was
that of focusing the electron beam. Around 1945 Leon Brillouin analyzed
what became known as Brillouin Flow in which a cylindrical electron beam
of
uniform charge density is confined by a uniform magnetic field. The
central electrons moves in a straight line; other electrons spiral around
the axis
with circumferential velocities that increase with radial distance. In a
note in the Physical Review in November, 1945, I pointed out that such
flow
could originate only from a cathode shielded from the magnetic field. When
the cathode is in the magnetic field, a field of greater strength is
needed
to confine a given electron current. Of my early traveling-wave tubes,
some had shielded cathodes and some didn't.
Producing a magnetic field to confine the electron beam by current in a
solenoid that surrounds the tube used power. Permanent magnets weren't
practical because they were very heavy. In trying to produce a uniform
field within the long, narrow beam one also produces a magnetic field that
extends far around the beam.
Around 1953 strong focusing revolutionized cyclotrons. In strong focusing,
a varying. rather than a uniform transverse magnetic field is used.
Various people tried to apply this directly to traveling-wave tubes. It
occurred to me that this missed the point; the fruitful procedure was to
use a longitudinal magnetic field but to change its direction periodically
along
the beam. Such a periodic magnetic field could be confined very close to
the axis, and it could be produced by comparatively light magnets. I
published a paper on this in the Journal of Applied Physics in September,
1953. Cal Quate produced an excellent realization of this idea in which
short ferrite tubes magnetized longitudinally were separated by thin
permalloy washers with central holes just larger than the glass envelope
of the traveling-wave tube.
I have described all my work on traveling-wave tubes that contributed
significantly to the amplifiers that have been manufactured and used in
microwave systems, including satellite systems. Much expert design and
development work went into the making of such tubes, but others did that
work.
I will discuss one other matter. That is my search for a satisfying
explanation of the mechanism of amplification in traveling-wave tubes. Not
just equations, but something physically appealing.
During my very early work I found a simple explanation of how electrons
traveling a little slower than an exponentially growing wave bunch in
regions so as to transfer power to the wave; I won't go through this
explanation.
Later I realized that, while like charges repel one another in a
"capacitive" medium such as space, they should attract one
another in and
"inductive" medium. In looking out at a helix while traveling a
little faster than the speed of a wave on the helix, one sees an inductive
impedance, so that at such a speed the electrons in the beam should
attract one another, in the longitudinal direction at least, and so bunch
together.
While these explanations were sound, they offered less insight than that
given by Lan Chu of MIT, but never, I think, published by him. Chu
observed
that one of the two principal space-charge waves on an electron beam, the
slower wave, has a negative energy. How can this be?
Because the beam itself has a positive kinetic energy. When a slow wave is
set up on the beam, this energy is reduced, and the stronger the wave the
greater the reduction in energy.
The explanation of the exponentially growing wave in traveling-wave tubes
is
simple. When the electromagnetic wave and the slow space-charge wave
have nearly the same velocity, there is a strong coupling between them.
This results in a wave that grows in amplitude at such a rate that the sum
of the negative energy of the slow space charge wave and the positive
energy of the electromagnetic wave remains constant.
The equations relating the amplitude and velocity of a wave that result
from the coupling of two waves are simple, and this new picture of the
operation of traveling-wave tubes made it easy to plot curves describing
the performance of the growing wave as various parameters were varied. I
had obtained the same curves earlier, but I felt that I now had a new
insight into them. I published this work in the Journal of Applied Physics
in February, 1954, and in more complete form in the Bell System Technical
Journal of November, 1954.
Coupled waves proved to be a powerful tool in understanding
non-propagating or "forbidden" regions in helices of large
diameter, through the coupling of forward and backward modes. Ping Tien
and I published a paper on this in the September 1954 issue of the
Proceedings of the IRE. The idea of modes and coupling of modes also
proved useful in understanding the behavior of wave-type parametric
amplifiers.
I find that I continued to publish papers concerning microwave tubes as
late as 1959, but my attention had turned elsewhere. In fact, I had always
had
wider interests, and by 1954 1 was pretty tired of traveling-wave tubes.
There was still a lot to be done, but it wasn't the sort of thing I could
do, or would want to.
About John R. Pierce.
John R. Pierce was born in Des Moines Iowa, March 27, 1910.
He graduated high school in Long Beach Calif. in 1929, after which
he attended Cal-Tech where he received his B.S. in 1933, his M.S. in 1934,
and Ph.D. in 1936.
John Pierce worked at Bell Telephone Laboratories for 35 years, during
which time he was involved in design work on a large number of
communications systems including Echo and Telstar. He retired in
1971.
During this time John Pierce had an active interest in authoring science
fiction articles for many of the popular magazines of the day. None of the
articles were in his name though, he used the pen name of John Roberts and
J.J. Coupling.
Now a Professor of Music Emeritus at the Center For Computer Research In
Music And Acoustics at Stanford University in California.
His recent book, co-authored with Michael Noll, Signals the Science of
Telecommunications, is available from The Scientific American Library.
|
|
|
|
|
|
|
|